Table of Contents (click to expand)
Traditional electricity is generated by the motion of free electrons, but neurons generate electric signals using the motion of ions across cell membranes.
Back in high school, as you open your biology textbook, you will almost undoubtedly see a chapter called “Central Nervous System” or “The Human Brain”. The lines read, “Neurons or nerve cells are the primary components of the nervous system. They send and receive information in the form of electrical signals from the sensory organs, facilitating communication with the brain.” The text probably also mentioned something about there being more than 86 billion neurons in every human brain.
However, the book likely didn’t explain how the neurons actually carried out this process of generating electrical signals. Yes, all of us remember the finger-like dendrites, axons and synapses, but the intricacies of the communication process may have eluded our high school minds.
Anatomy & Function Of Neurons
Before we get into the details of the constant chatter between body and brain, let’s review the basics of nerve cells. Neurons or nerve cells, along with glia, make up the central nervous system. Glia or glial cells are essentially Robin if neurons are Batman. They don’t directly participate in the exchange of information between neurons, but they do help define synaptic contacts and maintain the signaling abilities of neurons.
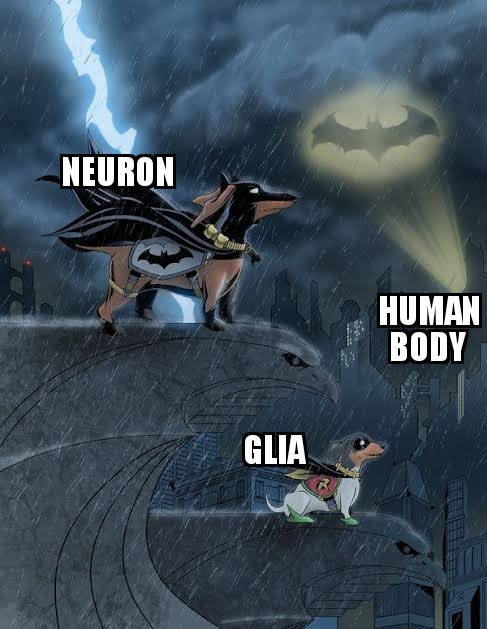
Neurons, on the other hand, are the Batman to the human body’s Gotham. Nothing in the human body happens without the neurons knowing about it. Just like every other cell, neurons have a cell body, often referred to as the soma, which contains the nucleus. Various branches or finger-like structures extend from opposite sides of the soma. An axon is the longer protrusion, while dendrites are the smaller branch-like structures on the other side of soma, as shown in the diagram below.
Neurons perform the critical task of carrying information across the entirety of the human body in three steps. First, they must receive signals or information from the sensory organs. If the signal is strong enough, it causes the neurons to transmit the signal to the next neuron by generating an action potential (see: electricity). Finally, this impulse reaches the target cells or other neurons.
Now, onto the important question that started this article… How is this communication achieved?
Also Read: What Is A Synapse? How Are Signals Transmitted Across It?
How Is Electricity Generated In Neurons?
The nerve cells transfer information by using both electrical and chemical signals. The electrical signals are used to move information within the nerve cells, whereas chemical signals are used to transfer information between two neighboring neurons.
Dendrites and the soma are responsible for receiving and processing all incoming information. A chemical substance called neurotransmitter binds to receptors on dendrites and causes conversion of the incoming chemical input into an electrical signal in the neuron, generating an action potential.
Each neuron receives multiple incoming signals from many cells at a time through different neurotransmitters. Excitatory neurotransmitters excite the next neuron by binding, whereas inhibitory chemicals inhibit the next neuron from firing. The input signal from multiple cells is “summated” in the cell body of the neuron and if the gross signal is excitatory, it will result in the next neuron firing, otherwise it doesn’t fire or is inhibited
The electric signal sent by the soma to the axon is called an action potential, which is a result of the change in membrane potential.
Let’s discuss this in greater detail, shall we?
Membrane Potential And Action Potential
To begin with, let’s take a quick chemistry lesson. Ions are charged particles that have either lost or gained electrons. When a particle loses an electron, it becomes positively charged and is called a cation. When a particle gains an electron, it becomes negatively charged and is called an anion.
Various ions float around in the human body. The difference in the net electrical charge of these ions on the inside and outside of the neuron is called the membrane potential. This difference in net electrical charge is due to the grouping of ions on opposite sides of the cell membrane.

In a rested state, sodium cations (Na+) and chloride anions (Cl-) are more prevalent outside the cell membrane of the neuron. On the inside of the cell membrane, however, potassium cations (K+) and various organic anions (A-) are present in greater numbers. The cell membrane of the nerve cell is selective in nature, only allowing some substances (ions) to pass through, while blocking the others. When in a rested state, only potassium cations (K+) can pass through the semipermeable membrane.
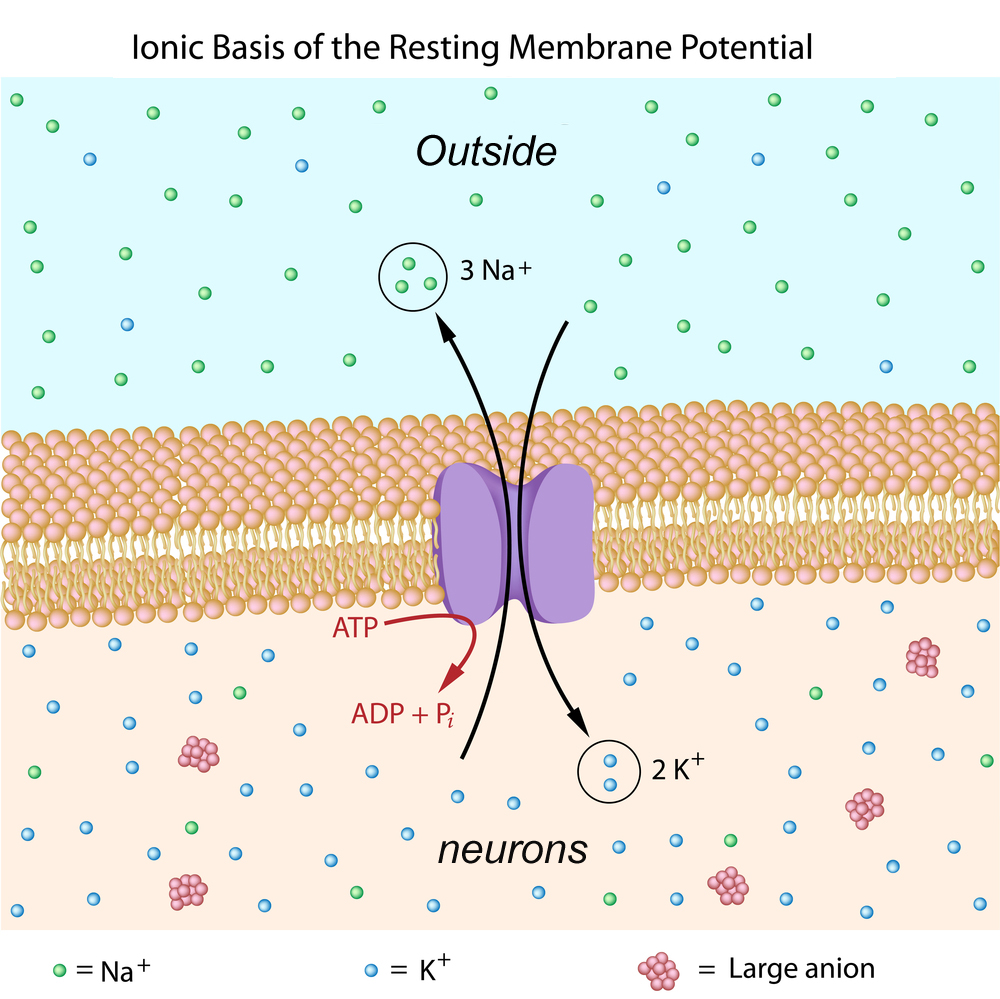
Thus, when at rest, the inside of the neuron is more negatively charged than the outside. This causes the resting membrane potential of a neuron to be around -70 mv. In simpler terms, the inside of the nerve cells is 70 mv less than the outside.
Depolarization
Resting membrane potential is affected when a stimulus (a signal from another neuron) is encountered. The stimulus or incoming signal causes the sodium channels of the membrane to open. Since the inside of the nerve cells is approximately -70mv, the positively charged sodium ions (Na+) start rushing in. The membrane potential, as a result, begins to drop below -70 mv, a process called depolarization.
-55 mv is the threshold value at which the neurons fire an action potential. Thus, when the membrane potential drops below -55 mv, an action potential is passed down the axon. An action potential is of the same kind/size and does not vary with the potential drop amount across the membrane.
Both the potassium and sodium cations are now present inside the neuron, causing the membrane potential to eventually drop to zero mv, and then even further! When the potential reaches the threshold of 30mV, it causes potassium channels to open, causing reversal of membrane potential.
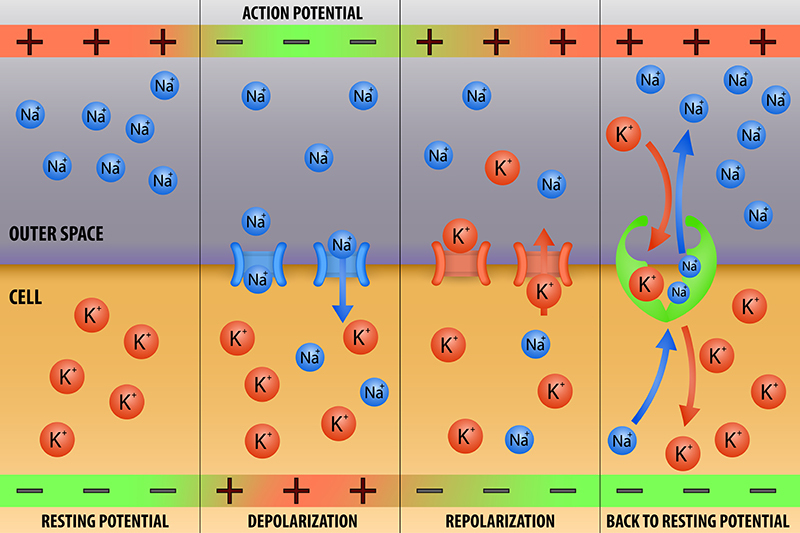
Repolarization & Hyperpolarization
Once the potassium channels open up, potassium cations (K+) start rushing out to reestablish balance. This process is called repolarization. Also, at around the same time, sodium channels start to close. This causes the membrane potential to return to the resting value of -70 mv.
The sodium channels close completely once the resting potential value is achieved. The potassium channels, however, take longer to close and the membrane potential shoots up to a value above -70 mv. The resting potential drops back down to -70 mv when the potassium channels close.
Also Read: Why Don’t Brain Cells Undergo Mitosis?
Conclusion
In short, this is how an electrical signal is generated in the neurons. Traditional electricity is generated by the motion of free electrons, but the electricity generated by neurons results from the motion of sodium and potassium ions across the cell membrane. The electrical signals only help to transfer information from the cell body through the axon to the synapse. The transfer of information between two different neurons, in most cases is facilitated by chemicals called neurotransmitters. In some other cases, the signals are passed from cell to cell directly through channels called ‘gap junctions’. Neurotransmitters are another fascinating topic that deserves an article all to itself: How do neurotransmitters work?
Do you remember how electricity is produced by neurons?
