Table of Contents (click to expand)
With the advent of technology for detecting neutrinos arriving from outer space, physicists have found a new way to observe our universe.
In a clear night sky with little ambient city light, we can see several celestial objects with the naked eye. Our eyes capture the photons coming from such objects, which our brains then interpret as an image.
In reality, the type of that photons our human eyes can detect is just a narrow band of frequencies out of the entire electromagnetic spectrum. This band is conveniently called “visible light”. Some other photon types have frequencies (or wavelengths) outside the visible band.
Astronomers have used photons outside the visible spectrum to take images of countless astronomical objects. This work includes using photons from radio waves (used by ALMA telescopes and Very Large Array observatory), infrared (used in Spitzer and WISE telescopes), X-ray (used by Chandra X-ray observatory) and gamma ray (Fermi Space Telescope) frequencies.
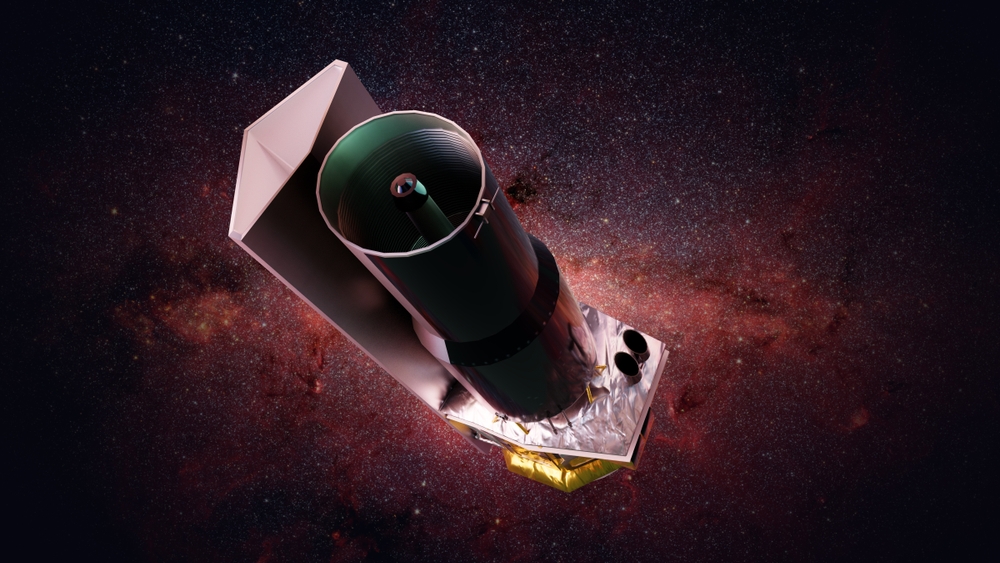
Using photons of different frequencies allows us to see the universe and its constituents that we cannot see in the visible light spectrum. There are instances when matter (especially very hot gases, black hole jets or cold matter) emits most of its radiation outside the visible light range. Due to this and several other advantages, astronomers work to obtain images of celestial objects in different wavelengths.
Photons Versus Neutrinos
The common element here is the observation of photons. However, in the past several decades, astronomers have tried to use particles other than photons to observe space, and one of these candidate particles are neutrinos.
In June 2023, astronomers at the IceCube observatory, located at the South Pole, successfully managed to obtain a map of neutrino emissions in the Milky Way galaxy. While physicists have long thought it was possible to use neutrinos for observations, scientists first accomplished this feat only recently.
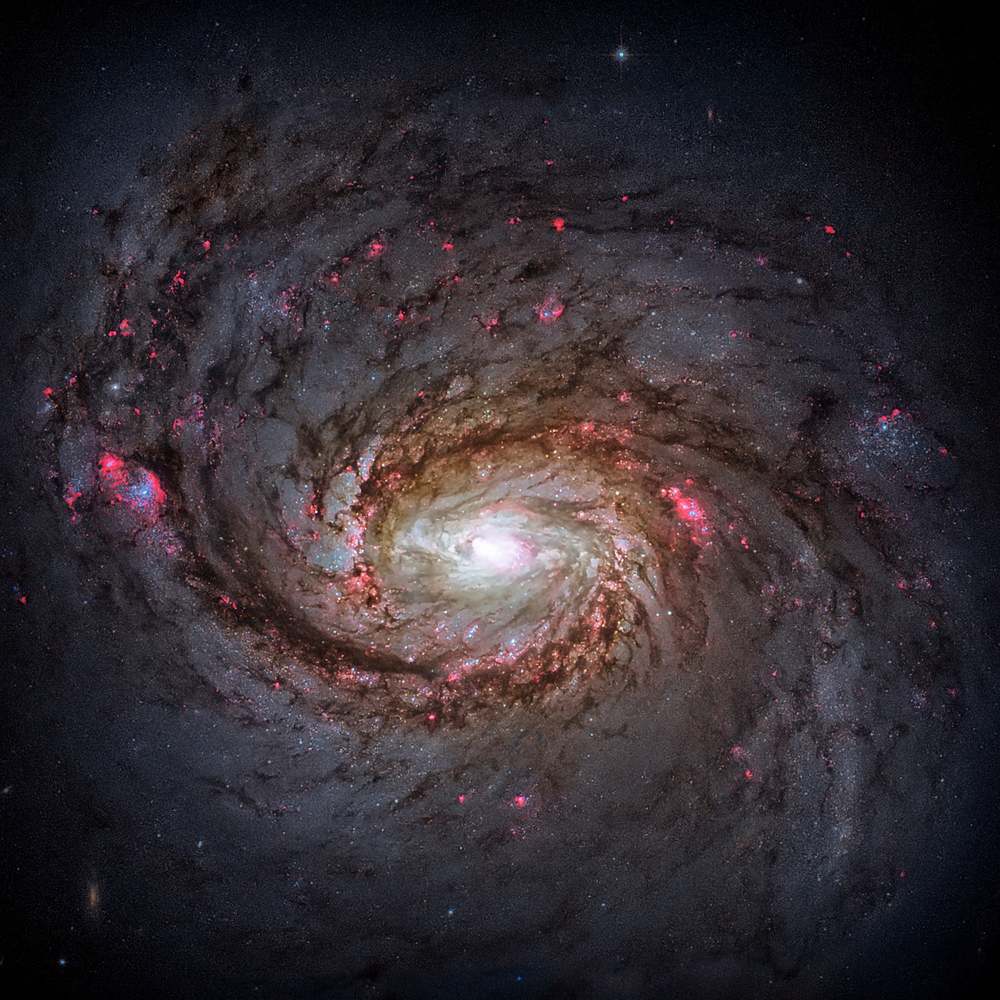
In 2018, the IceCube observatory detected highly energetic neutrinos from a blazar around 4 billion light-years away. In November 2022, it detected neutrino emissions from a galaxy designated NGC 1068, also called Messier 77. Astronomers found that these neutrinos were coming from its core, where they think there is an active black hole with a mass that is greater than the black hole at the center of our own galaxy.
And now, we can observe our home galaxy using particles other than photons.
One common way in which neutrinos form is via cosmic rays. Cosmic rays form near extreme regions of the universe, like near neutron stars and black holes. Here, when matter gets accreted into such objects due to their strong gravity, some release of energy happens. This energy would then accelerate protons or other nuclei that may be present there and race away into outer space.
Sometimes, these accelerated particles could interact with other matter or electromagnetic radiation. This usually occurs near the neutron star or the black hole itself. When this interaction happens, the result is either gamma rays or high-energy neutrinos. These are the neutrinos that astronomers attempt to observe.
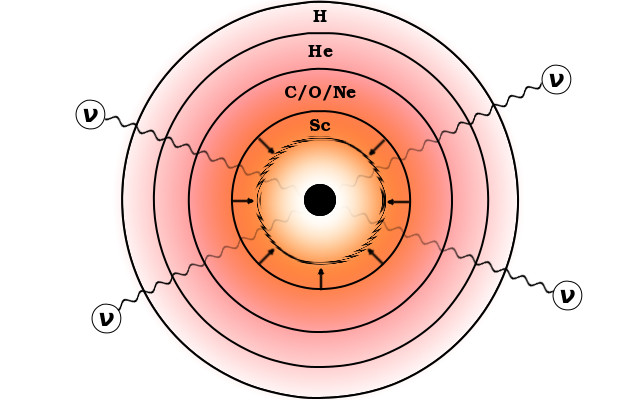
Also, neutrinos are produced during events like the death of stars, including supernovae. Scientists think they also got generated in less than one second after the Big Bang.
The reason astronomers try to use neutrinos as a way to observe the universe is that a large number of them can pass through objects uninterrupted. They have no electric charge and have a small rest mass. Thus, they only weakly interact with matter and radiation, and are mostly uninterrupted during their journey to Earth.
This can help us directly find the location of their source and obtain information about its formation.
Astronomers think neutrinos would possess essential information on astronomical events, like the death of a star. However, we cannot see them with our naked eyes, and due to their nature, neutrinos are generally hard to detect.
Also Read: What Particle Are We Searching For In The Antarctic Ice?
Using Neutrinos To Observe Outer Space
Fortunately, scientists have devised clever methods to track the presence of neutrinos. One of the most prominent among these detectors is the IceCube neutrino observatory. As its name suggests, this observatory uses ice to detect neutrinos.

Some of the neutrinos coming from space interact with the atomic nuclei present in the ice. Due to this interaction, the production of Cherenkov radiation occurs, which is detected by optical sensors placed at the bottom of the ice layer. This detection comes in two forms: track and cascade.
Tracks usually appear as an elongated straight line of photons, allowing us to find their direction of origin more easily. Tracks arise through muons that are produced, either from cosmic ray reactions in the Earth’s atmosphere or neutrinos interacting with nuclei.
Cascades are short-range particle showers, generally happening due to the scattering of neutrinos with ice nuclei. Cascades appear as a small, spherical signal. While one drawback is that their direction of motion is difficult to obtain, cascade events are fully contained within the observatory, allowing for a complete measurement of their energy.

Scientists obtained an image of the neutrino map of the Milky Way galaxy using cascade neutrinos. However, since finding the direction of these events is nearly impossible, an obvious question arises: how do we know that these detected neutrinos are from the Milky Way galaxy?
Secondly, there is also a massive number of background neutrinos arising from the interactions of cosmic ray particles with the Earth’s atmosphere. As a result, a method is needed to separate the neutrinos arriving from outer space (especially the ones from the Milky Way galaxy) and those that originated in the Earth’s atmosphere.
For this, scientists employed machine learning and artificial intelligence algorithms to solve the two issues. Using data collected from neutrinos detected over the past ten years, the algorithm ‘learnt’ their properties to determine which neutrinos came from the atmosphere and which came from outer space. By filtering out those neutrinos originating from the atmosphere, a map of the neutrino emissions in the Milky Way galaxy could be produced.
Also Read: How Far Back Into The Past Can We See In The Night Sky?
Prospects Of Neutrino Astronomy
So why is this neutrino map significant? One could say that it provides a new way to observe the universe. There is more weight to this statement than our ability to to detect neutrinos.
The ability to detect neutrinos would provide more hidden details about outer space that electromagnetic radiation simply cannot give us. The neutrino map of the Milky Way galaxy tells us that our galaxy is a bright source of neutrinos. While knowing their exact origins is one thing, an important point we can take from this is that there is more out there to see than what photons can illuminate.
One reason we should observe both neutrinos and photons in observational astronomy is that photons get scattered by the interstellar and intergalactic medium. This is particularly true for the gamma rays produced alongside neutrinos during cosmic ray interactions. Thus, observing these neutrinos would be one way of learning about astrophysical phenomena that we might not detect with photons.

Observing neutrinos would therefore be an addition to the area of multi-messenger astronomy. Multi-messenger astronomy refers to using different particles to observe the universe. Using electromagnetic radiation is one way, but a more recent addition is the detection of gravitational waves. Now, neutrino astronomy is yet another addition. With this, we can observe many astrophysical events in multiple ways, giving a clearer “picture”.
Neutrino astronomy could answer questions like the exact origin of individual neutrinos. While we broadly know their sources, there is no way to figure out the precise origin of each neutrino. They might also hold clues about dark matter. Scientists think that neutrinos could form from dark matter colliding with itself. Prospects like this make the pursuit of neutrino astronomy even more exciting!
How well do you understand the article above!

References (click to expand)
- Kovalev, Y. Y., Plavin, A. V., & Troitsky, S. V. (2022, November 29). Galactic Contribution to the High-energy Neutrino Flux Found in Track-like IceCube Events. The Astrophysical Journal Letters. American Astronomical Society.
- IceCube Collaboration*†, Abbasi, R., Ackermann, M., Adams, J., Aguilar, J. A., Ahlers, M., … Zhelnin, P. (2023, June 30). Observation of high-energy neutrinos from the Galactic plane. Science. American Association for the Advancement of Science (AAAS).
- K Wright. Milky Way Viewed through Neutrinos - Physics (APS). American Physical Society
- IceCube neutrinos give us first glimpse into the inner depths of .... Consin–Mad
- Antarctic Experiment Maps the Milky Way Galaxy's High .... Columbia University
- Our galaxy seen through a new lens: neutrinos ... - IceCube. Consin–Mad
- F Halzen. (2021) [2110.01687] The Observation of High-Energy Neutrinos .... arXiv
- What is multi-messenger astronomy? - UW–Madison News. Consin–Mad
- In a First, Scientists See Neutrinos Emitted by the Milky Way. Scientific American