Table of Contents (click to expand)
Since the discovery of crystallography in 1912, many crystals and their geometric details have been studied, but nothing has created a divide in the scientific community quite like quasicrystals. Their forbidden symmetry and aperiodic arrangement has opened a whole new world of materials for us.
On April 8th, 1982, Dan Shechtman, an Israeli scientist, was working alone in a laboratory at the National Bureau of Standards (now NIST) in Maryland. He was studying his material samples under a transmission electron microscope (TEM) when he saw something impossible—an unbelievable vision, a creature?
He tried a number of different techniques to re-verify what he had observed, but there was no mistake. The substance he discovered clearly exhibited properties prohibited by the laws of physics.
In fact, he had stumbled upon something that would change the world’s perception of matter forever. Dan Shechtman had discovered Quasicrystals—the forbidden matter.
More specifically, he discovered something that shouldn’t exist… but does!
Why Isn’t It A Crystal?
As the name suggests, they are “almost” crystals, but not exactly so, in the traditional sense. To understand why they’re not crystals, first we need to know what is a crystal.
A crystal is a substance with a regular arrangement of atoms that are ordered and periodic. They are formed by the assembly of basic repeating structures known as the unit cells. Imagine a Rubik’s cube as a single crystal, and all those tiny squares as the unit cells.
The unit cells repeat themselves in 3 dimensions and give rise to a network known as the crystal lattice. This lattice determines the shape or geometry of a crystal. To learn more about crystals and crystal systems, click here.
Most of us know that solids are either crystals or amorphous powders, so does that mean quasicrystals are amorphous? No, they’re not. In amorphous materials, the arrangement of atoms or molecules is completely random and irregular.
In Quasicrystalline materials, the arrangement of atoms or molecules is ordered and aperiodic. The atomic structures are arranged in a pattern such that there are no gaps between the structures, but no pattern ever repeats itself. The structures repeat themselves throughout the pattern, but in completely different orientations every time.
The first quasicrystalline material discovered was a solidified Aluminum and Manganese (10-14%) alloy. The electron diffraction pattern revealed the icosahedral, 5-fold rotational geometry of the material. This forbidden symmetry of the crystal gave it the tag of being “impossible”.
Also Read: How Is Crystal Structure Determined?
Why Was It Considered Impossible?
You have likely noticed that the words “forbidden symmetry”, “ordered”, and “periodic” keep popping up in the text. That’s because these words hold the essence of what makes a quasicrystal so special. To understand what these words mean in this context, we need to pay attention to dimensions and shapes.
Let’s begin with what makes a form of symmetry “forbidden”. Imagine that you have tiles of 5 different shapes with different rotational symmetries: 1) a rectangle with 2-fold rotational symmetry (i.e., if you rotate a rectangle in a plane there will be two instances where the shapes look identical); 2) a triangle with 3-fold symmetry; 3) a square with 4-fold symmetry; 4) a pentagon with 5-fold symmetry; and 5) a hexagon with 6-fold symmetry.
Our task is to tile the walls with these shapes, leaving no gap in between.
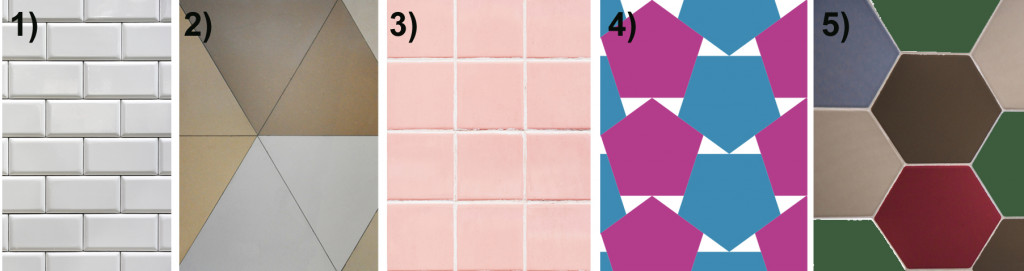
We can see that the shapes 1, 2, 3, and 5 can easily be placed with no gaps between the tiles, but that’s not the case for tile 4, the pentagon. No matter how you rotate the tiles, there is no way to pack them without leaving a gap between. The angles simply don’t fit into each other.
This is why a molecular or atomic structure having 5-fold rotational symmetry or any number higher than 6 was considered forbidden in the formation of a crystal, because a crystal requires shapes that can pack without a gap. A quasicrystal tackles this problem with an ordered, but aperiodic arrangement, similar to Penrose tiles.
Now, let’s try to understand what we mean by ordered, periodic, and quasi-periodic (or aperiodic) in all three dimensions.
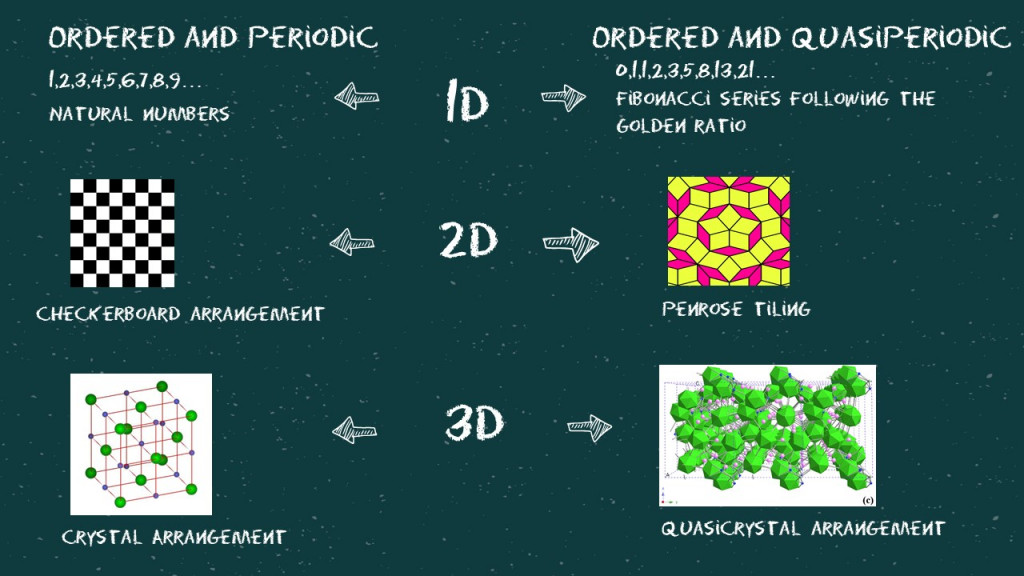
The mathematics behind this is really fascinating. A structure loses its periodicity when projected on a lower dimension. If we lived in a 2D world where we were all stick figures, the ordered and periodic 3D structure of an NaCl crystal would be considered aperiodic. Similarly, somewhere in a 6D world, quasiperiodic crystals are considered perfectly ordered and periodic.
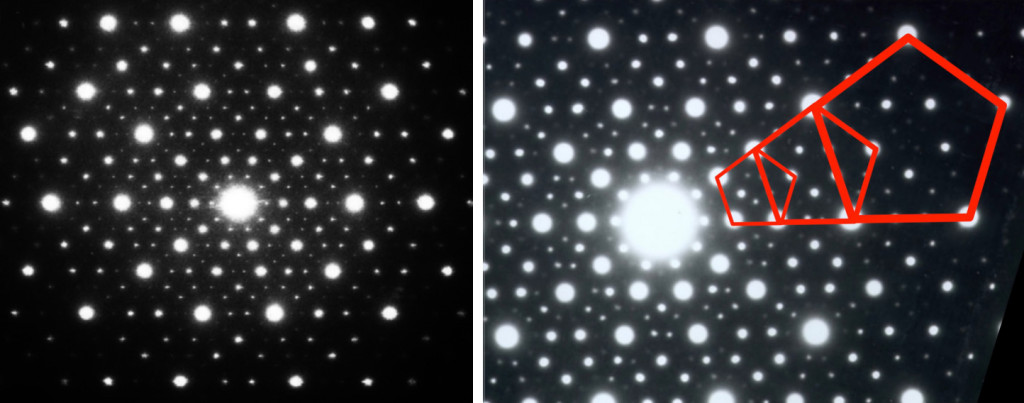
If you look closely at the diffraction pattern and try to connect the bright dots (as shown in the image), you will find three pentagons of different sizes. The sizes of these pentagons follow the golden ratio or the Fibonacci series. Looks like everything is connected after all!
Also Read: What Are Time Crystals?
What Makes It Different?
Not only the symmetry form, but many other parameters for what makes it different from the crystal could exist. One of them could be how such a quasicrystal grows.
During the self-assembly or growth of a crystal, the atoms interact with their neighbors like a game of telephone. One atom passes a message to the next atom on how and where to form a bond. This template growth gives rise to a regular network.
However, as per simulations run by Glotzer and Engels at the University of Michigan, the atoms in quasicrystals also interact with each other, but not with the very next one. They interact with ones that are three atoms away from them. Then each particle nucleates at the center, growing outwards and creating a dendritic fashion like the Penrose tiling.
Even though quasi-periodicity sounds very exotic, it isn’t very rare. There are many aluminum and other metallic alloys that are made of stable quasiperiodic crystals. Most of these are synthetically obtained, but scientists have found three naturally occurring quasicrystals from a piece of rock that fell from space. The Khatyrka meteorite in Russia houses all the natural QCs discovered to date.
Why Are Quasicrystals Important?
Quasicrystals (QCs) have unique electronic, optical, and mechanical properties. The QCs might be brittle on their own, but can reinforce steel and make it as strong as armor. Due to its non-stick and scratch-resistant properties, they can also be used to coat utensils instead of Teflon. They have a low coefficient of friction and could be used in the synthesis of superfluids (fluids with zero to negligible velocity).
QCs could even be used to improve camouflaging materials. What we see is dependent on how light bounces off the body. The spatial arrangement of atoms in icosahedral crystals could potentially help in manipulating light so as to hide the actual physical appearance of an object. Someday we may actually have a disappearing Helicarrier from The Avengers films!
The discovery of QCs also redefined what was meant by the term “crystal”. In 1994, the International Union of crystallographers defined a crystal as “A solid having an essentially discrete diffraction pattern”. This redefinition finally made room for quasiperiodic crystals.
Conclusion
What makes quasicrystals beautiful isn’t just their intricate microstructure, but also the sheer obstinance of their discoverer. For years he was the recipient of skepticism from the scientific community. Many of his initial papers were rejected by publication journals. After his work was finally published in 1984, it created a ripple in the scientific community. Some praised his work, while many others remained cynical about his findings.
Even big names in chemistry, such as Linus Pauling, went on record to say that “Danny Shechtman is talking nonsense. There are no quasicrystals, just quasi-scientists”. Pauling, to the day he died, tried to disprove the existence of QCs.
Dan Shechtman’s faith in his work opened up new and exciting doors for scientists to uncover the existence of even more such amazing and impossible materials. His discovery also led him to win the 2011 Nobel prize in Chemistry!
How well do you understand the article above!

References (click to expand)
- The DiSCovery of QuaSiCrySTalS - Nobel Prize. The Nobel Prize
- Shechtman, D., Blech, I., Gratias, D., & Cahn, J. W. (1984, November 12). Metallic Phase with Long-Range Orientational Order and No Translational Symmetry. Physical Review Letters. American Physical Society (APS).
- KTHLearningLab (2012). Nobel Lecture: "The Discovery of Quasicrystals". Youtube
- Growth Rules for Quasicrystals - LIPN. Sorbonne Paris North University
- Quasicrystals Are Nature's Impossible Matter - VICE. Vice
- (2007) How do Quasicrystals Grow? - University of Michigan. glotzerlab.engin.umich.edu
- M Senechal. WHAT IS...a Quasicrystal. The American Mathematical Society
- Quasicrystals - an overview | ScienceDirect Topics. ScienceDirect