‘The Demon Core’ was a 6.2 kg subcritical mass of plutonium core built during the 1940s to be used in nuclear weapons. The core resulted in the death of two scientists, which earned it the strange and sinister nickname.
In the 1940s, during the Second World War, the United States of America developed three radioactive plutonium cores to be used in their nuclear bombs. Among these, the first was used in the Trinity Nuclear Test, the world’s first nuclear explosion. The second core was the heart of the ‘Fat Man’ nuclear bomb dropped over the city of Nagasaki, Japan.
The third was supposed to be used in another bomb, which was to be dropped over the city of Tokyo. However, Japan surrendered following the detonations at Hiroshima and Nagasaki, and the war came to an end.
Thus, the third bomb had no immediate use, and its core, named ‘Rufus’ was sent to the Los Alamos Laboratory in New Mexico for research. Here, it received the nickname ‘The Demon Core’. So, how did ‘Rufus’ become ‘The Demon Core’? Let’s find out!
What Is The Demon Core?
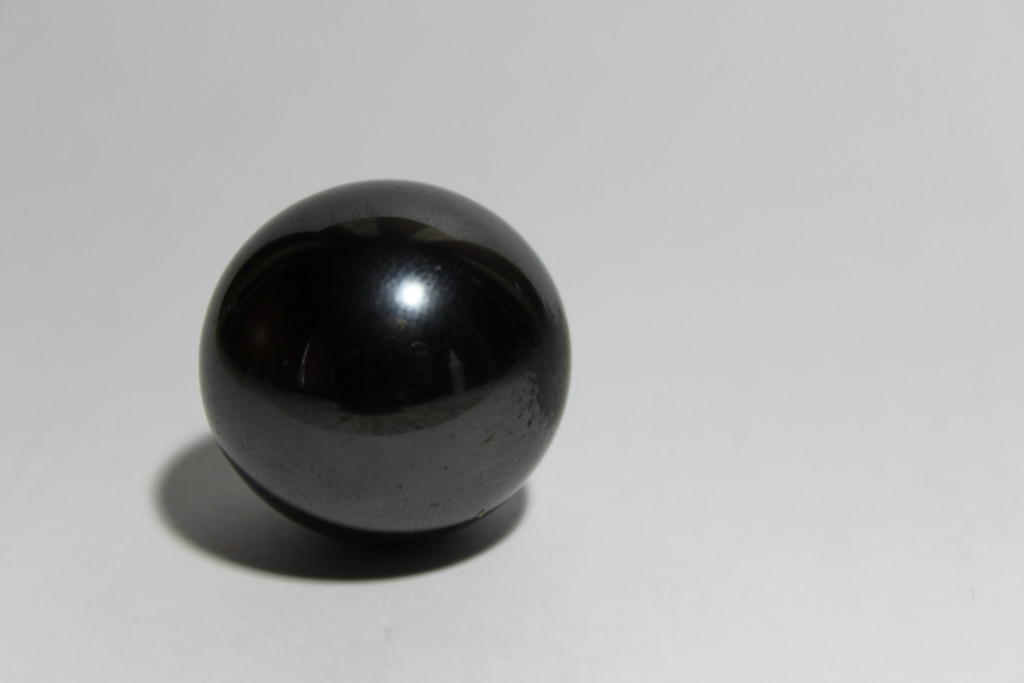
The Demon core is a 6.2 kg Plutonium-Gallium sphere with a diameter of 8.9 cm (smaller than a basketball). The Plutonium-239 isotope used in the core is a manmade element produced in nuclear reactors. It is highly unstable, radioactive, and decays by emitting alpha particles, which is damaging to our tissues.
Hence, the core has a nickel coating, which blocks the short-range alpha particles and also prevents the core from rusting. Furthermore, due to radioactivity, the core is warm to the touch.
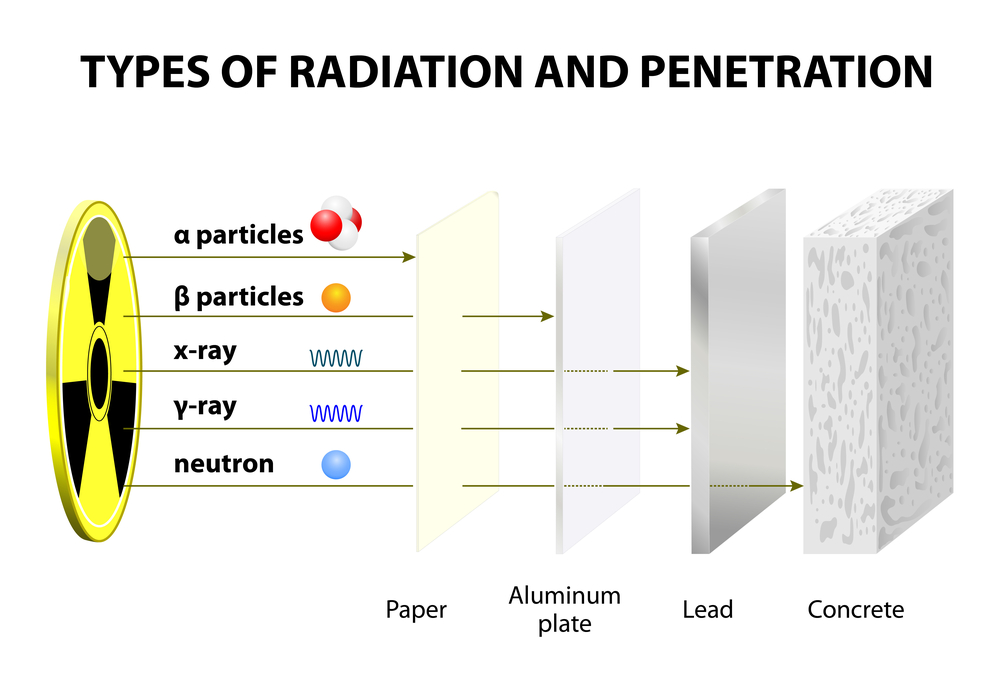
The core works on the principle of Nuclear Fission. Pu-239 is a fissile element. That is, upon absorbing a neutron, the Pu-239 nucleus splits into two smaller nuclei by releasing a large amount of energy, along with the release of 2- 3 extra neutrons. These neutrons are then absorbed by other Pu nuclei, and the fission continues. This results in a nuclear chain reaction.
The core is built in such a way that it doesn’t go into a chain reaction immediately by itself, but only when we need it to.
Also Read: What Exactly Is Radioactivity ?
What Is The ‘Super-Critical’ State?
Fissile materials exist in three states; sub-critical, critical and super-critical.
If the Pu atoms are arranged in such a way that, on average, each fission event does not necessarily trigger another fission event, the system is Sub-Critical. A chain reaction does not occur in this state, and the fission simply dies out.
On average, if each fission reaction triggers exactly ‘one’ new fission reaction, the system becomes Critical and initiates a steady chain reaction. Nuclear reactors work on this principle.
Finally, on average, if each fission reaction triggers more than one fission reaction, the system gets Super-Critical. This results in an exponentially growing chain reaction.
However, the fission rate does not go all the way to infinity. The rate of fission keeps increasing until the system finally reaches its limit. After some time, due to the excessive amount of energy, the system blows itself apart, and the reaction ceases. The cores of nuclear bombs are based on this principle.
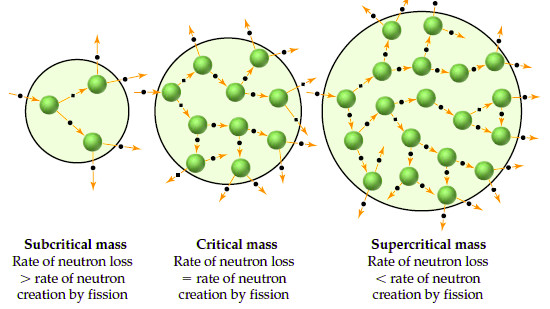
Thus, in the core, plutonium must be maintained below a certain mass, called the Critical Mass. It is the minimum amount of fissile material required to sustain a chain reaction. Once plutonium reaches its critical mass, a fission chain reaction initiates. However, there is a catch. As the name suggests, critical mass doesn’t depend on ‘mass’ alone.
For a given mass of plutonium, critical mass can be attained by manipulating other factors, such as the concentration, density or purity of the material. In other words, criticality can be achieved by increasing the plutonium-neutron interaction. Let’s discuss this in a bit deeper detail.
How To Attain Criticality?
There are different ways to attain criticality of a given mass of Plutonium.
1. Increasing The Concentration Of Plutonium
The closer the plutonium atoms get, the higher their chances are of colliding with a neutron. Hence, a higher concentration leads to criticality.
Many factors help us achieve a close-packed arrangement of Pu atoms. These include:
- Shape: A spherical shape is ideal for atoms to be closer. For example, Gallium helps to hold Plutonium in a spherical shape.
- Density: If the density is high, there will be a large number of atoms packed into a small volume.
- Purity: The purer the material, the higher the concentration of Pu atoms.
2. Reducing The Loss Of Neutrons
In a fission reaction, not all the released neutrons are captured by other nuclei. Some of the fast-moving neutrons leak or escape without undergoing further reaction. The use of neutron reflectors solves this issue. They reflect the neutrons back into the system and help to attain criticality without changing the mass of plutonium.
Criticality Experiments
In the Los Alamos Laboratory, scientists started criticality experiments to see how far the core could go before reaching criticality. They used neutron reflectors to assess the efficiency of the core. The Demon Core was 5% below criticality, meaning that even a small trigger could initiate the nuclear chain reaction.
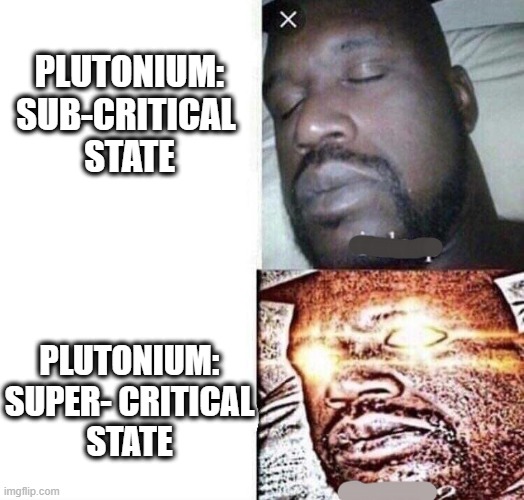
Criticality experiments are extremely dangerous. Once the core goes critical, fission reaction initiates, and a deadly amount of energy and neutron radiation is emitted — more than enough to kill anyone in its vicinity.
Considering the dangers involved, famous Physicist Richard Feynman reportedly described the experiment as “Tickling a Sleeping Dragon’s Tail”. His words later became true. Within two years, two prominent scientists succumbed to the dragon’s fire.
Also Read: What Does The Fallout From Atomic Testing Tell Us About Our Moon?
The First Victim: Harry Daghlian
In 1945, American physicist Harry K. Daghlian started performing criticality experiments. He placed Tungsten Carbide bricks around the core to reflect the neutrons into it. He tried out different numbers of bricks and different orientations to see what would bring the core closest to criticality.
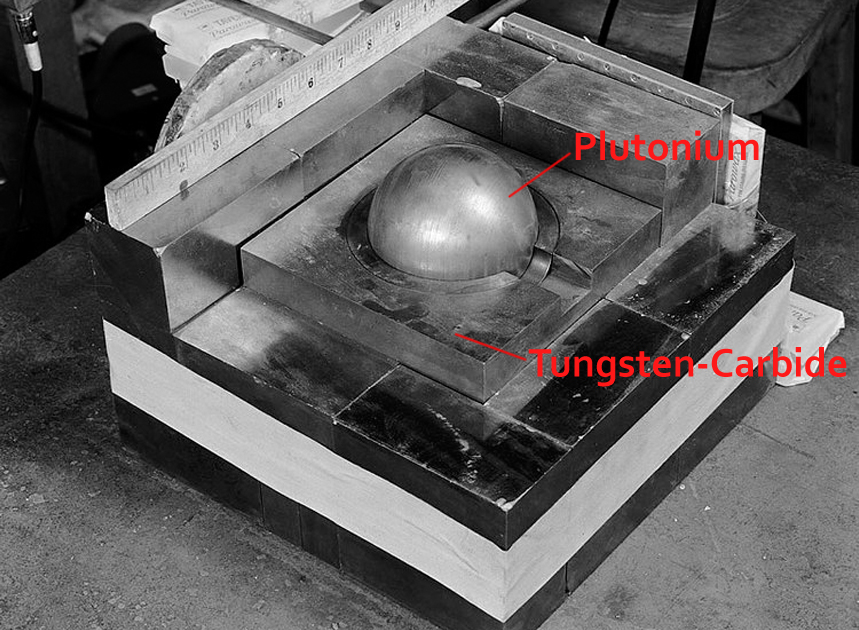
However, curiosity got the best of him. He tried testing the core to its maximum limit. On August 21, 1945, when he was about to place one last brick, the counter indicated that placing one more brick would make the core go “super-critical”. At that point, he carefully tried to remove the brick, but accidentally DROPPED IT!
The dragon was awake!
There was a bright flash of blue light and a surge of heat as the brick fell over the core. Daghlian received about 500 rads of radiation, which is much higher than the maximum limit of 200rem. He immediately took the brick back, but those seconds of exposure had already dug his grave. Soon, he went into a coma and died 25 days later of radiation exposure. This was the first death due to a criticality experiment.
The Second Victim: Louis Slotin
On May 21, 1946, the Demon core claimed its second victim, Louis Slotin—a physicist who was Daghlian’s co-worker in the Manhattan Project. Coincidentally, he passed away on another 21st at the same hospital as his colleague.
After Daghlian’s death, Slotin continued with criticality experiments, but instead of bricks, he used two Beryllium half-spheres as neutron reflectors.
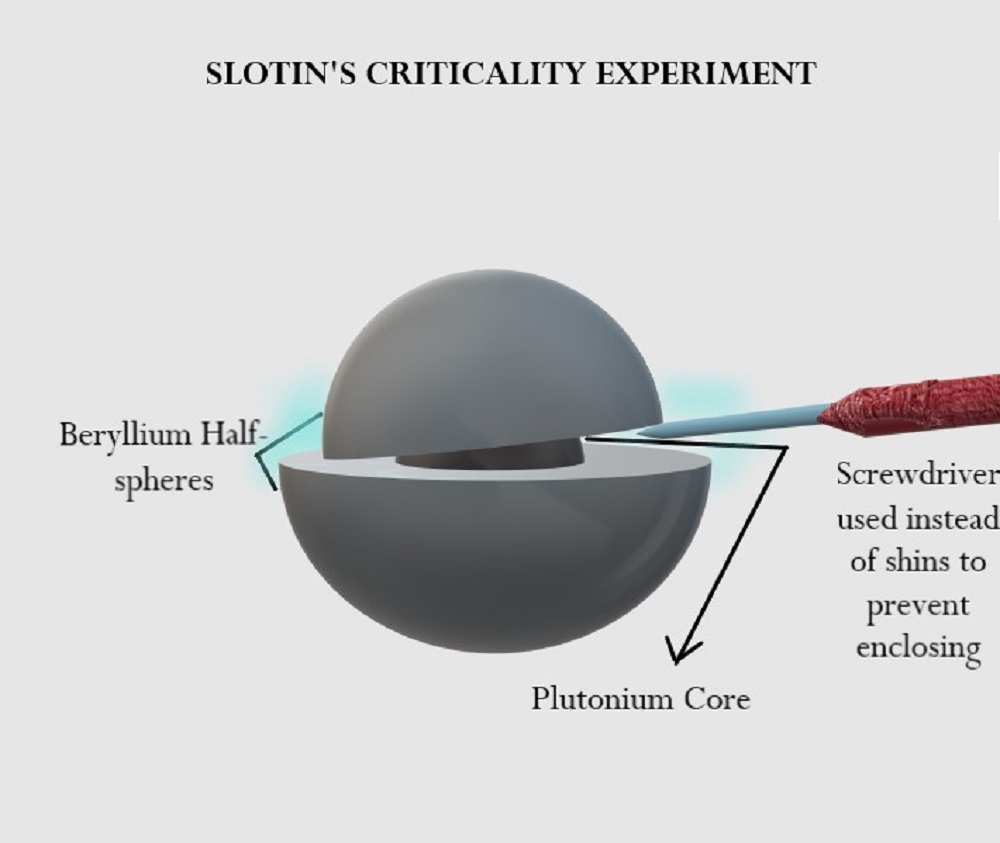
He would place the core inside the bottom hemisphere and slowly lower the top sphere over it. Lowering the sphere would increase neutron reflection, and lead to criticality. However, if the outer sphere totally enclosed the core, complete neutron reflection would cause the core to go “super-critical”. Therefore, the experiment required placing two wedges between the hemispheres to prevent it from closing fully.
Slotin was brilliant, but reckless, and instead of the wedges, he simply used the tip of a flathead screwdriver to prevent the hemispheres from enclosing the core. He would tilt the screwdriver to adjust the sphere and note the change in criticality. On the 21st of May, he was performing a similar experiment with 7 other colleagues when THE SCREWDRIVER SLIPPED! The shell enclosed the core and it went “super-critical”.
There was a similar heatwave and blue light. Slotin immediately tipped off the sphere, but he was already exposed to a deadly amount of radiation (1000 rads). Nine days later, he passed away due to radiation illness. According to the doctors, his body had experienced something similar to a “3-Dimensional Sunburn”.
Conclusion
The criticality experiments indirectly resulted in the death of other people, including scientists and security guards present during the incident. The deaths of two prominent scientists sparked a debate about the precautions and safety measures that should be followed during scientific experiments. As a result, the government halted criticality experiments, given the risks involved. Also, the Plutonium core received its nickname “Demon Core”.
It is mind-boggling to think that a small metal sphere can be this dangerous. It proves how strange and amazing the universe really is. The 1989 movie “Fat Man and Little Boy” portrays a visual representation of the core and the criticality accident. After the incidents, authorities melted down the demon core and recast it to build new cores. Thus, the core met its end and became part of a new era of weaponry and experimentation.
Also Read: What Were The Differences Between Little Boy And Fat Man?
How well do you understand the article above!

References (click to expand)
- A new subcritical measurement tool will help scientists protect and preserve the nation’s stockpile. - www.lanl.gov
- Costa, D. A., Cournoyer, M. E., Merhege, J. F., Garcia, V. E., & Sandoval, A. N. (2017, May 1). A primer on criticality safety. Journal of Chemical Health & Safety. American Chemical Society (ACS).
- The Atomic Bomb and the End of World War II. The National Security Archive
- CDC Radiation Emergencies | Radioisotope Brief: Plutonium .... The Centers for Disease Control and Prevention
- Rodriguez, Y. R. (2014). Plutonium. Encyclopedia of Toxicology. Elsevier.
- Harry Daghlian - Nuclear Museum - Atomic Heritage Foundation. The Atomic Heritage Foundation
- Louis Slotin - Nuclear Museum - Atomic Heritage Foundation. The Atomic Heritage Foundation