Table of Contents (click to expand)
Niel Bohr’s Atomic Theory states that – an atom is like a planetary model where electrons were situated in discretely energized orbits. The atom would radiate a photon when an excited electron would jump down from a higher orbit to a lower orbit. The difference between the energies of those orbits would be equal to the energy of the photon.
Niels Bohr was a Danish physicist and is considered one of the founding fathers of quantum mechanics, precisely old quantum mechanics. For his exemplary contributions to science, the Carlsberg brewing company decided to give him a house situated right next to one of their breweries. The house was connected to the brewery by a pipeline. Bohr was rewarded with a lifetime supply of free beer that would pour out of a tap at his whim. What extraordinary feat did Niels Bohr accomplish to deserve this prestigious honor, and well, a Nobel Prize?
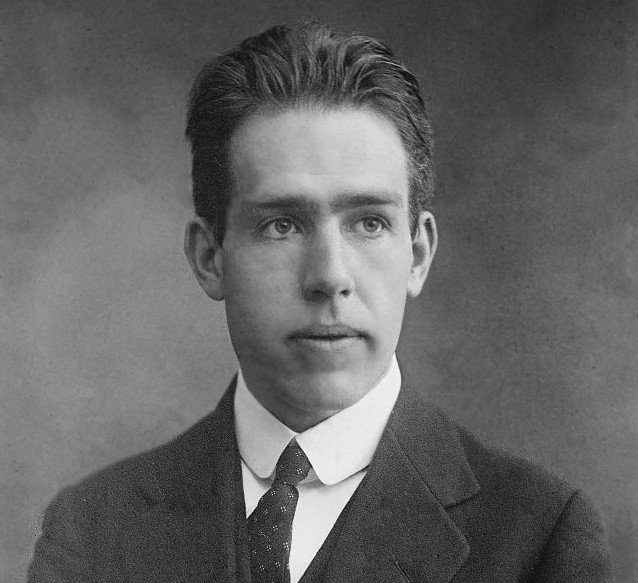
Quite simply, Niels Bohr illuminated the mysterious inner-workings of the atom. Although he arrived at his model and its principles in collaboration with the august founder of the atomic nucleus, Ernest Rutherford, the model is only credited to Bohr. Originally called the Rutherford-Bohr atomic model, it is now commonly referred to as Bohr’s atomic model.
To understand Bohr’s theory, we must first understand what prior discoveries led him to pursue his revolutionary ideas.
Rutherford’s Failed Model
It was Sir J.J. Thomson who first discovered that the atom wasn’t indivisible after all, a notion believed to be true for centuries. However, the subatomic particle he discovered was negatively charged. If atoms were merely a cluster of negative charges, then chairs, tables, you and I would be anything but stable. He immediately realized that to account for matter’s stability, there must be a net positive charge to neutralize the negativity.
Thomson devised what became the very first model of an atom. He suggested that the negative particles, which he called electrons, were like seeds embedded in a positively charged watermelon. The model is popularly known as the plum or raisin pudding model. I’m sure the analogy is obvious.
This view held true until Ernest Rutherford showed that when positive particles are shot at an atom, most of them pass straight through, but a few are observed to be deflected at a large angle. Rutherford realized that most of the atom was filled with empty space, but at the center was a dense, point-like concentration of positive charge. He called this the atom’s nucleus. The volume of empty space between an atom’s electrons and its nucleus is so huge that if the atom were expanded to the size of a baseball stadium, its nucleus would be the size of a baseball.
Rutherford suggested that perhaps the atomic system was analogous to our Solar System, where the electrons revolve around the nucleus like planets revolving around the Sun. The crucial difference was, of course, that the electrons were captivated by electrostatic force, rather than gravity. However, Maxwell and Hertz would have vehemently disagreed.
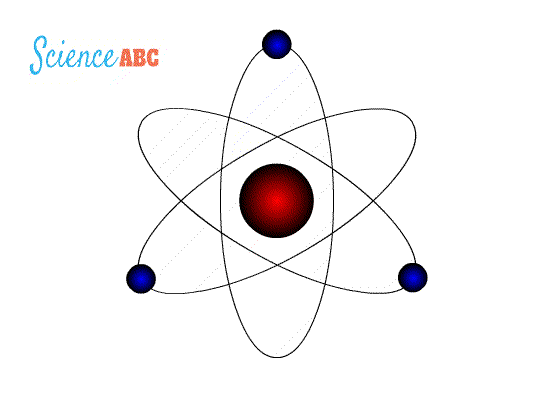
Maxwell’s laws of electromagnetism had recently established that the motion of a charged particle, such as an electron, comes at the expense of energy. Thus, a revolving electron, like the circus men on motorbikes racing around inside a sphere, would soon spiral and collapse as it ran out of fuel. In fact, physicists calculated that it would take just 16 picoseconds for an electron to radiate all its energy and collapse into its nucleus. That is one-trillionth of a second. A new atomic model that would explain matter’s profound stability had yet to be discovered.
Also Read: What Is J.J. Thomson’s Plum Pudding Model?
The Hydrogen Spectrum
Another absurdity that perplexed physicists at that time was Planck’s black body radiation and the “emission spectrum” given off by different atoms. The word ‘spectrum’ was first coined by Newton to describe the rainbow of colors that sprang from his prism.
Similarly, when a body is heated, it radiates a spectrum of electromagnetic energy. If you burn a bar of iron with a blowtorch, you will observe that as the temperature of the bar increases, the color it assumes will also change gradually. First, it’s red, then orange, and then bright white before veering towards violet.
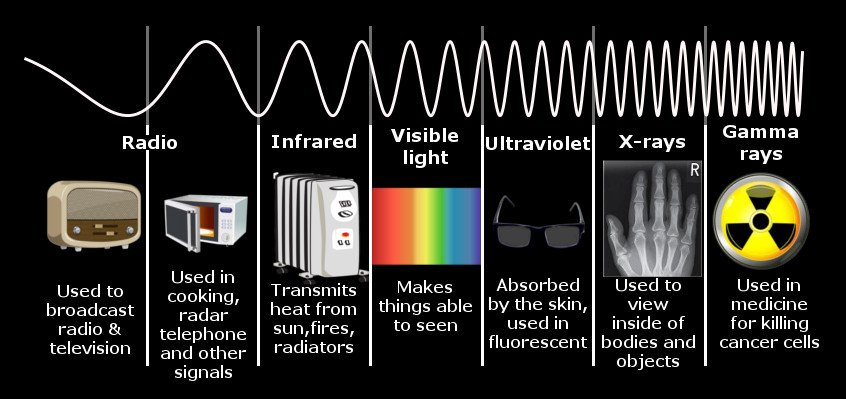
This is because the electromagnetic energy radiated by that iron bar falls in the range of visible light – light that our eyes can detect. If you were to heat the bar to 20,000 Kelvin, the energy radiated would be in the ultraviolet (UV) range. In fact, every object in the Universe radiates such a spectrum of energy, including human beings, but since the temperature of our body is so low, the energy emitted is also meager, somewhere in the range of infrared light. Our eyes are equipped with sensors that can only identify one member amongst the several members of the electromagnetic spectrum.
Max Planck called this phenomenon black body radiation. If you were to plot the heat’s intensity with the wavelength of light radiated, you would observe a peak at a certain range of wavelengths. The peak for the Sun’s core burning at 6,000K lies partly in the visible range, while for a star burning at 20,000K, it lies completely in the UV range, and for a stellar explosion, such as the birth of a black hole, it lies in the gamma range.
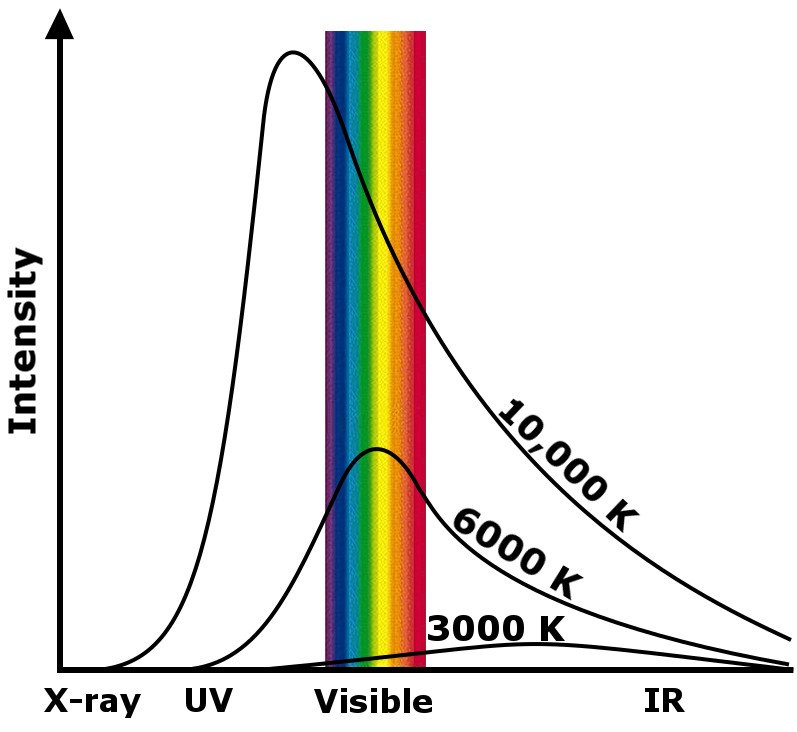
Furthermore, the graph depicts that as the temperature of a body declines, the wavelength of light it radiates increases. For instance, the radiation from the Big Bang may have started out as gamma rays, but as it cooled down over more than 13 billion years, the wavelengths elongated to microwaves. If you were to plot these waves on a black background, you would witness a beautiful, hazy mélange of colors – a continuous spectrum.

However, the major implication of Planck’s finding was that the radiated energy traveled in discrete packets, like rigid particles, which Einstein later called photons. The energy of a single quantum is inversely proportional to its wavelength or directly proportional to its frequency. With a fundamental constant of proportionality called Planck’s constant, h, the energy E for a frequency v can be expressed as E = hv.
Now, if you were to heat a volume of gas of a single element in this way and plot the colors on a black background, you would observe something of an anomaly. The spectrum is no longer a beautiful or continuous mixture of colors. Instead, it comprises a series of definite, single-colored lines intermittently separated by chunks of the absolutely black background. For instance, take a look at the ever-famous spectrum of hydrogen.

In fact, each and every element in the Universe paints its own unique, discontinuous spectrum. While hydrogen’s spectrum lies in the visible range, certain elements produce a spectrum that lies in the ultraviolet or infrared range. For this reason, an element’s spectrum is considered its fingerprint. The knowledge of its uniqueness allows us to study the composition of stars and has even aided scientists in discovering new elements!
Looking at the spectrum of hydrogen, it was obvious that only certain colors appeared because only certain frequencies – those associated with these colors – were radiated. Given that, why would atoms exhibit this peculiar behavior? What atomic structure would restrict them so severely to express themselves so laconically? Niels Bohr, in 1913, finally realized why.
Bohr’s Atomic Model
Bohr went ahead with Rutherford’s Solar System model, but added a small tweak. He rectified its failing aspect by suggesting (for a reason yet to be known) that electrons revolve around a nucleus in fixed or definite orbits. He claimed that in these orbits, the electrons wouldn’t lose any energy, therefore ensuring that they didn’t collapse into the nucleus.
Bohr called these fixed orbits “stationary orbits”. He claimed that the orbits weren’t randomly situated, but were instead at discrete distances from the nucleus in the center, and that each of them was associated with fixed energies. Inspired by Planck’s theory, he denoted the orbits by n, and called it the quantum number.
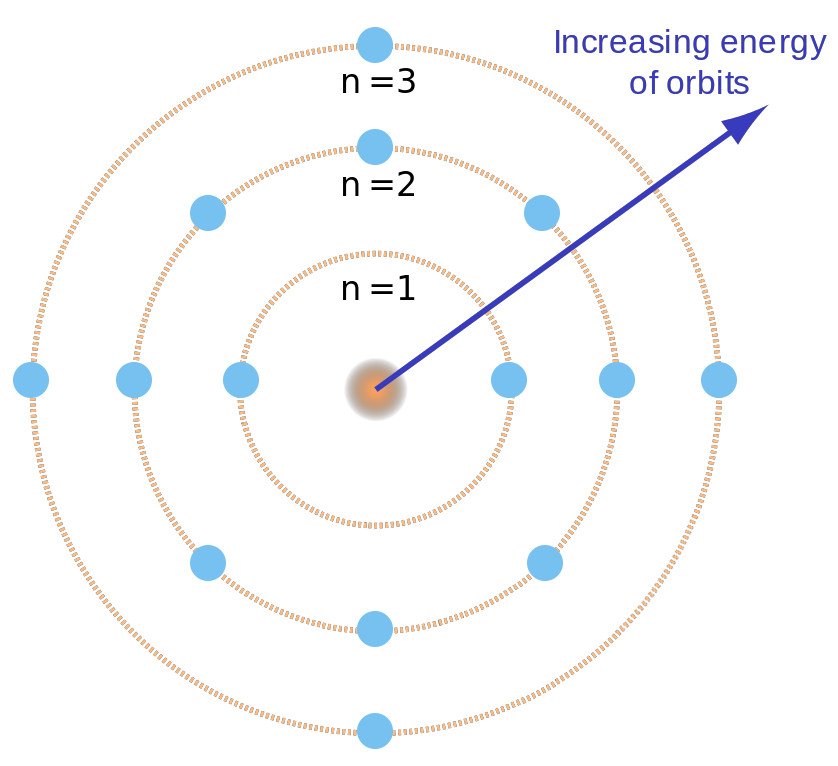
However absurd the theory might have appeared, it predicted the spectrum of hydrogen splendidly. According to it, when a gas is heated, its energized electrons jump from an orbit of lower energy to an orbit of higher energy (in the case of hydrogen, from n =1 to n = 2). However, to regain stability, they must jump back down to the lower energy orbits. While undergoing this transition, the electron must lose some of its energy, and it is this energy that is radiated in the form of light!
The discrete nature of orbits provides a concise explanation for the discrete nature of photons. Bohr found that the energy of an emitted photon is equal to the difference of energies of the two levels between which the electron makes its jump. For instance, infrared is radiated when the electron makes a short leap, while ultraviolet is radiated when it makes a much larger leap. This relation can be simply expressed as E2 – E1 = hv. Conversely, an electron jumps to a higher orbit when it absorbs a photon.
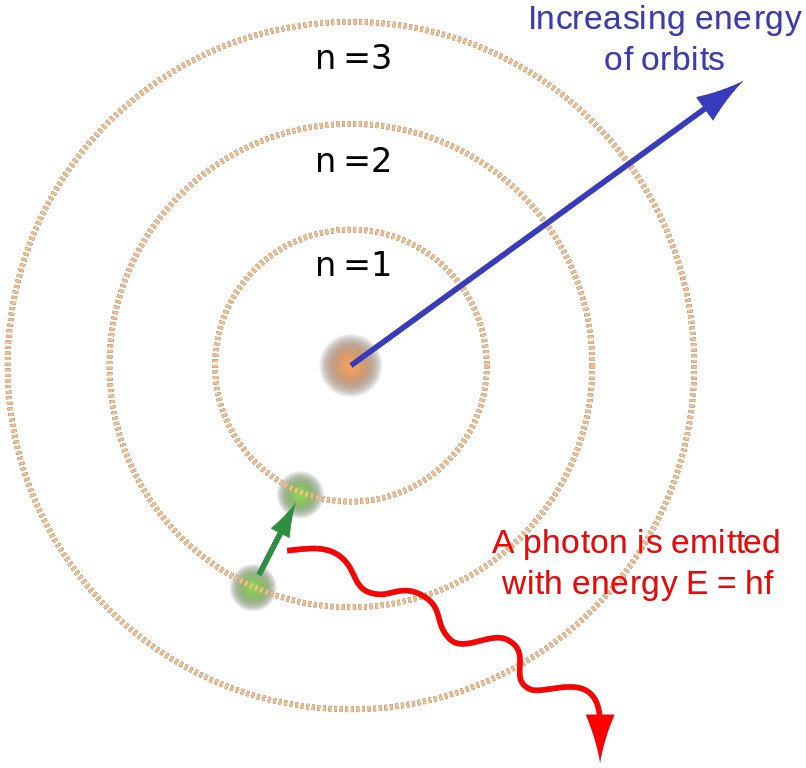
The spectrum of an atom is restricted to particular colors because its concrete, organized structure allows its electrons to only certain energy transitions – and therefore certain frequencies of light. Now, if an atom of hydrogen only contains a single electron, why does its spectrum consist of multiple colors? Well, this is because the gas is composed of millions and billions of atoms with electrons raised to different orbits that are higher or lower than those nearby.
So, this was Bohr’s model – a planetary model where electrons were situated in discretely energized orbits. The atom would radiate a photon when an excited electron would jump down from a higher orbit to a lower orbit. The difference between the energies of those orbits would be equal to the energy of the photon.
Also Read: Protons And Electrons Have Opposite Charges, So Why Don’t They Pull On Each Other?
Shortcomings
Unfortunately, Bohr’s model could only explain the behavior of a system where two charged points orbited each other. This meant the hydrogen atom, in particular. It also included ionized helium (helium has two electrons, so ionization would seize one of those, leaving it with only one) or double-ionized lithium (lithium has three electrons… you do the math). His theory couldn’t explain the behavior of any other atom except hydrogen.
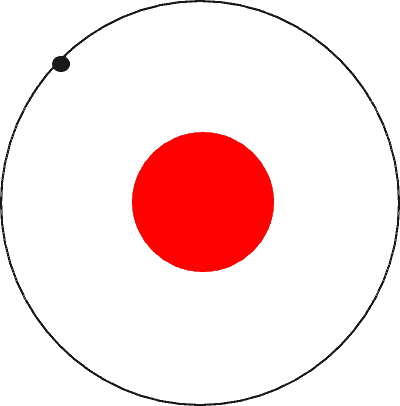
Furthermore, his theory dictated that electrons align in the stationary orbits like beads on a thread, meaning that he had assumed a non-interactive system of electrons. This horribly discounts the violently repulsive electrostatic force between not just two, but multiple electrons clustered together that would thrust each other miles away. Eventually, we discovered that electrons do not just revolve, but also rotate or spin on their axis. Bohr’s model couldn’t explain why this didn’t lead to a loss of energy.
It is speculated that part of the reason why Bohr’s theory was so readily accepted is that it made successful theoretical predictions of multiple spectra that hadn’t been observed. Still, it is widely lauded, as it revolutionized modern physics by paving the way for modern quantum mechanics. Eventually, modern quantum mechanics perfectly explained the true nature of energy shells, how electrons would inhabit each of them, as well as the problem of spin.
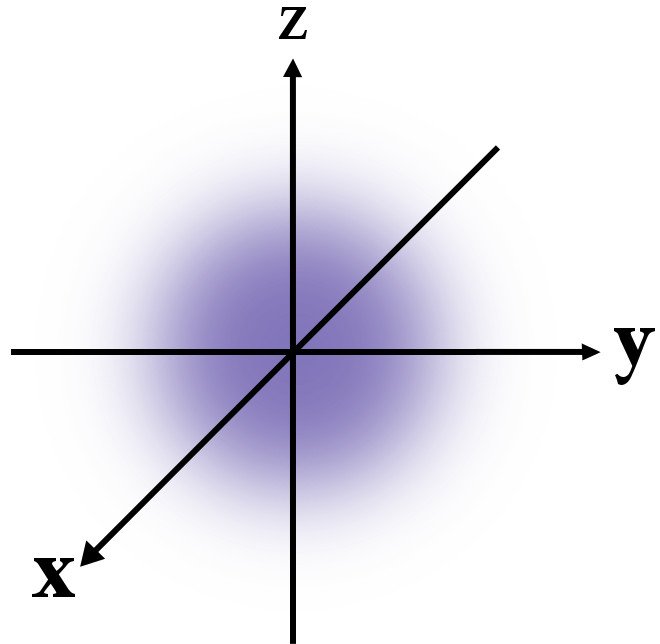
However, for its simplicity, Bohr’s ideas still continue to exist and dominate high school physics. The textbooks are replete with concentric circles filled with electrons surrounding a nucleus, which resembles the beads-in-a-thread model. For his contribution, Bohr surely deserved that free beer after all. And of course… a Nobel Prize.
How well do you understand the article above!
